Estimating somatic mutation rates by bottlenecked duplex sequencing in non-model organisms: Daphnia magna as a case study
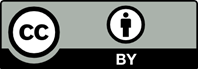
Somatic mutations are evolutionarily important as determinants of individual organismal fitness, as well as being a focus of clinical research on age-related disease, such as cancer. Identifying somatic mutations and quantifying mutation rates, however, is extremely challenging and genome-wide somatic mutation rates have only been reported for a few model organisms. Here, we describe the application of Duplex Sequencing on bottlenecked WGS libraries to quantify somatic nuclear genome-wide base substitution rates in Daphnia magna. Daphnia, historically an ecological model system, has more recently been the focus of mutation studies, in part because of its high germline mutation rates. Using our protocol and pipeline, we estimate a somatic mutation rate of 5.6 × 10-7 substitutions per site (in a genotype where the germline rate is 3.60 × 10-9 substitutions per site per generation). To obtain this estimate, we tested multiple dilution levels to maximize sequencing efficiency and developed bioinformatic filters needed to minimize false positives when a high-quality reference genome is not available. In addition to laying the groundwork for estimating genotypic variation in rates of somatic mutations within D. magna, we provide a framework for quantifying somatic mutations in other non-model systems, and also highlight recent innovations to single molecule sequencing that will help to further refine such estimates.
1. Kinde I, Wu J, Papadopoulos N, Kinzler KW, Vogelstein B. Detection and quantification of rare mutations with massively parallel sequencing. Proc Natl Acad Sci USA. 2011 Jun;108(23):9530–5. https://doi.org/10.1073/pnas.1105422108 PMID:21586637
2. Hiatt JB, Pritchard CC, Salipante SJ, O’Roak BJ, Shendure J. Single molecule molecular inversion probes for targeted, high-accuracy detection of low-frequency variation. Genome Res. 2013 May;23(5):843–54. https://doi.org/10.1101/gr.147686.112 PMID:23382536
3. Salk JJ, Schmitt MW, Loeb LA. Enhancing the accuracy of next-generation sequencing for detecting rare and subclonal mutations. Nat Rev Genet. 2018 May;19(5):269–85. https://doi.org/10.1038/nrg.2017.117 PMID:29576615
4. Singh RR. Next-Generation Sequencing in High-Sensitive Detection of Mutations in Tumors: Challenges, Advances, and Applications. J Mol Diagn. 2020 Aug;22(8):994–1007. https://doi.org/10.1016/j.jmoldx.2020.04.213 PMID:32480002
5. Abbasi A, Alexandrov LB. Significance and limitations of the use of next-generation sequencing technologies for detecting mutational signatures. DNA Repair (Amst). 2021 Nov;107:103200. https://doi.org/10.1016/j.dnarep.2021.103200 PMID:34411908
6. Schmitt MW, Kennedy SR, Salk JJ, Fox EJ, Hiatt JB, Loeb LA. Detection of ultra-rare mutations by next-generation sequencing. Proc Natl Acad Sci USA. 2012 Sep;109(36):14508–13. https://doi.org/10.1073/pnas.1208715109 PMID:22853953
7. Kennedy SR, Schmitt MW, Fox EJ, Kohrn BF, Salk JJ, Ahn EH, et al. Detecting ultralow-frequency mutations by Duplex Sequencing. Nat Protoc. 2014 Nov;9(11):2586–606. https://doi.org/10.1038/nprot.2014.170 PMID:25299156
8. Hoang ML, Kinde I, Tomasetti C, McMahon KW, Rosenquist TA, Grollman AP, et al. Genome-wide quantification of rare somatic mutations in normal human tissues using massively parallel sequencing. Proc Natl Acad Sci USA. 2016 Aug;113(35):9846–51. https://doi.org/10.1073/pnas.1607794113 PMID:27528664
9. Matsumura S, Sato H, Otsubo Y, Tasaki J, Ikeda N, Morita O. Genome-wide somatic mutation analysis via Hawk-Seq™ reveals mutation profiles associated with chemical mutagens. Arch Toxicol. 2019 Sep;93(9):2689–701. https://doi.org/10.1007/s00204-019-02541-3 PMID:31451845
10. You X, Thiruppathi S, Liu W, Cao Y, Naito M, Furihata C, et al. Detection of genome-wide low-frequency mutations with Paired-End and Complementary Consensus Sequencing (PECC-Seq) revealed end-repair-derived artifacts as residual errors. Arch Toxicol. 2020 Oct;94(10):3475–85. https://doi.org/10.1007/s00204-020-02832-0 PMID:32737516.
11. Abascal F, Harvey LM, Mitchell E, Lawson AR, Lensing SV, Ellis P, et al. Somatic mutation landscapes at single-molecule resolution. Nature [Internet]. 2021 Apr 28 [cited 2021 Apr 29]; Available from: http://www.nature.com/articles/s41586-021-03477-4 https://doi.org/10.1038/s41586-021-03477-4
12. Kivioja T, Vähärautio A, Karlsson K, Bonke M, Enge M, Linnarsson S, et al. Counting absolute numbers of molecules using unique molecular identifiers. Nat Methods. 2011 Nov;9(1):72–4. https://doi.org/10.1038/nmeth.1778 PMID:22101854.
13. Kennedy SR, Salk JJ, Schmitt MW, Loeb LA. Ultra-sensitive sequencing reveals an age-related increase in somatic mitochondrial mutations that are inconsistent with oxidative damage. PLoS Genet. 2013;9(9):e1003794. https://doi.org/10.1371/journal.pgen.1003794 PMID:24086148.
14. Sloan DB, Broz AK, Sharbrough J, Wu Z. Detecting Rare Mutations and DNA Damage with Sequencing-Based Methods. Trends in Biotechnology; Oxford. 2018 Jul;36(7):729–40. https://doi.org/10.1016/j.tibtech.2018.02.009.
15. Jabara CB, Jones CD, Roach J, Anderson JA, Swanstrom R. Accurate sampling and deep sequencing of the HIV-1 protease gene using a Primer ID. Proc Natl Acad Sci USA. 2011 Dec;108(50):20166–71. https://doi.org/10.1073/pnas.1110064108 PMID:22135472.
16. Ho EK, Macrae F, Latta LC 4th, McIlroy P, Ebert D, Fields PD, et al. High and Highly Variable Spontaneous Mutation Rates in Daphnia. Mol Biol Evol. 2020 Nov;37(11):3258–66. https://doi.org/10.1093/molbev/msaa142 PMID:32520985.
17. Kluettgen B, Duelmer U, Engels M, Ratte HT. ADaM, an artificial freshwater for the culture of zooplankton. Water Res. 1994 Apr;28(3):743–6. https://doi.org/10.1016/0043-1354(94)90157-0.
18. Li H, Durbin R. Fast and accurate short read alignment with Burrows-Wheeler transform. Bioinformatics. 2009 Jul;25(14):1754–60. https://doi.org/10.1093/bioinformatics/btp324 PMID:19451168.
19. Li H. Aligning sequence reads, clone sequences and assembly contigs with BWA-MEM. arXiv. 2013 March; arXiv:1303.3997. https://doi.org/10.48550/arXiv.1303.3997
20. Danecek P, McCarthy SA. BCFtools/csq: haplotype-aware variant consequences. Bioinformatics. 2017 Jul;33(13):2037–9. https://doi.org/10.1093/bioinformatics/btx100 PMID:28205675.
21. Danecek P, Bonfield JK, Liddle J, Marshall J, Ohan V, Pollard MO, et al. Twelve years of SAMtools and BCFtools. Gigascience. 2021 Feb;10(2):giab008. https://doi.org/10.1093/gigascience/giab008 PMID:33590861.
22. Smit, AF. RepeatMasker [Internet]. 2013. Available from: http://www.repeatmasker.org
23. Smit AF, Hubley R. RepeatModeler [Internet]. 2008. Available from: http://www.repeatmasker.org
24. Quinlan AR, Hall IM. BEDTools: a flexible suite of utilities for comparing genomic features. Bioinformatics. 2010 Mar;26(6):841–2. https://doi.org/10.1093/bioinformatics/btq033 PMID:20110278.
25. Thorvaldsdóttir H, Robinson JT, Mesirov JP. Integrative Genomics Viewer (IGV): high-performance genomics data visualization and exploration. Brief Bioinform. 2013 Mar;14(2):178–92. https://doi.org/10.1093/bib/bbs017 PMID:22517427.
26. Stoler N, Arbeithuber B, Povysil G, Heinzl M, Salazar R, Makova KD, et al. Family reunion via error correction: an efficient analysis of duplex sequencing data. BMC Bioinformatics. 2020 Mar;21(1):96. https://doi.org/10.1186/s12859-020-3419-8 PMID:32131723.
27. Milholland B, Dong X, Zhang L, Hao X, Suh Y, Vijg J. Differences between germline and somatic mutation rates in humans and mice. Nat Commun. 2017 May;8(1):15183. https://doi.org/10.1038/ncomms15183 PMID:28485371.
28. Supek F, Lehner B. Scales and mechanisms of somatic mutation rate variation across the human genome. DNA Repair (Amst). 2019 Sep;81:102647. https://doi.org/10.1016/j.dnarep.2019.102647 PMID:31307927
29. Keith N, Jackson CE, Glaholt SP, Young K, Lynch M, Shaw JR. Genome-Wide Analysis of Cadmium-Induced, Germline Mutations in a Long-Term Daphnia pulex Mutation-Accumulation Experiment. Environ Health Perspect. 2021 Oct;129(10):107003–10. https://doi.org/10.1289/EHP8932 PMID:34623885
30. Arbeithuber B, Hester J, Cremona MA, Stoler N, Zaidi A, Higgins B, et al. Age-related accumulation of de novo mitochondrial mutations in mammalian oocytes and somatic tissues. PLoS Biol. 2020 Jul;18(7):e3000745. https://doi.org/10.1371/journal.pbio.3000745 PMID:32667908