Measurement of skeletal muscle glucose uptake in mice in response to acute treadmill running
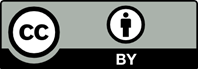
Skeletal muscle contractions stimulate glucose uptake into the working muscles during exercise. Because this signaling pathway is independent of insulin, exercise constitutes an important alternative pathway to increase glucose uptake, also in insulin-resistant muscle. Therefore, much effort is being put into understanding the molecular regulation of exercise-stimulated glucose uptake by skeletal muscle. To delineate the causal molecular mechanisms whereby muscle contraction or exercise regulate glucose uptake, the investigation of genetically manipulated rodents is necessary. Presented here is a modified and optimized protocol assessing exercise-induced muscle glucose uptake in mice in response to acute treadmill running. Using this high-throughput protocol, running capacity can accurately and reproducibly be determined in mice, and basal- and exercise-stimulated skeletal muscle glucose uptake and intracellular signaling can precisely and dose-dependently be measured in awake mice in vivo without the need for catheterization and with minimal loss of blood.
1. Sylow L, Kleinert M, Richter EA, Jensen TE. Exercise-stimulated glucose uptake - regulation and implications for glycaemic control. Nat Rev Endocrinol. 2017 Mar;13(3):133–48. https://doi.org/10.1038/nrendo.2016.162 PMID:27739515
2. Hoffman NJ, Parker BL, Chaudhuri R, Fisher-Wellman KH, Kleinert M, Humphrey SJ, et al. Global Phosphoproteomic Analysis of Human Skeletal Muscle Reveals a Network of Exercise-Regulated Kinases and AMPK Substrates. Cell Metab. 2015 Nov;22(5):922–35. https://doi.org/10.1016/j.cmet.2015.09.001 PMID:26437602
3. Wang D, Zhong L, Nahid MA, Gao G. The potential of adeno-associated viral vectors for gene delivery to muscle tissue. Expert Opin Drug Deliv. 2014 Mar;11(3):345–64. https://doi.org/10.1517/17425247.2014.871258 PMID:24386892
4. Bengtsson NE, Hall JK, Odom GL, Phelps MP, Andrus CR, Hawkins RD, et al. Muscle-specific CRISPR/Cas9 dystrophin gene editing ameliorates pathophysiology in a mouse model for Duchenne muscular dystrophy. Nat Commun. 2017 Feb;8(1):14454. https://doi.org/10.1038/ncomms14454 PMID:28195574
5. Gurumurthy CB, Lloyd KC. Generating mouse models for biomedical research: technological advances. Dis Model Mech. 2019 Jan;12(1):dmm029462. https://doi.org/10.1242/dmm.029462 PMID:30626588
6. Fueger PT, Bracy DP, Malabanan CM, Pencek RR, Wasserman DH. Distributed control of glucose uptake by working muscles of conscious mice: roles of transport and phosphorylation. Am J Physiol Endocrinol Metab. 2004 Jan;286(1):E77–84. https://doi.org/10.1152/ajpendo.00309.2003 PMID:13129858
7. Maarbjerg SJ, Jørgensen SB, Rose AJ, Jeppesen J, Jensen TE, Treebak JT, et al. Genetic impairment of AMPKalpha2 signaling does not reduce muscle glucose uptake during treadmill exercise in mice. Am J Physiol Endocrinol Metab. 2009 Oct;297(4):E924–34. https://doi.org/10.1152/ajpendo.90653.2008 PMID:19654283
8. Halseth AE, Bracy DP, Wasserman DH. Overexpression of hexokinase II increases insulinand exercise-stimulated muscle glucose uptake in vivo. Am J Physiol. 1999 Jan;276(1):E70–7. https://doi.org/10.1152/ajpendo.1999.276.1.E70 PMID:9886952
9. Jeppesen J, Maarbjerg SJ, Jordy AB, Fritzen AM, Pehmøller C, Sylow L, et al. LKB1 regulates lipid oxidation during exercise independently of AMPK. Diabetes. 2013 May;62(5):1490–9. https://doi.org/10.2337/db12-1160 PMID:23349504
10. Sylow L, Nielsen IL, Kleinert M, Møller LL, Ploug T, Schjerling P, et al. Rac1 governs exercise-stimulated glucose uptake in skeletal muscle through regulation of GLUT4 translocation in mice. J Physiol. 2016 Sep;594(17):4997–5008. https://doi.org/10.1113/JP272039 PMID:27061726
11. Sylow L, Møller LL, Kleinert M, D’Hulst G, De Groote E, Schjerling P, et al. Rac1 and AMPK Account for the Majority of Muscle Glucose Uptake Stimulated by Ex Vivo Contraction but Not In Vivo Exercise. Diabetes. 2017 Jun;66(6):1548–59. https://doi.org/10.2337/db16-1138 PMID:28389470
12. Fentz J, Kjøbsted R, Birk JB, Jordy AB, Jeppesen J, Thorsen K, et al. AMPKα is critical for enhancing skeletal muscle fatty acid utilization during in vivo exercise in mice. FASEB J. 2015 May;29(5):1725–38. https://doi.org/10.1096/fj.14-266650 PMID:25609422
13. Sylow L, Møller LL, Kleinert M, Richter EA, Jensen TE. Stretch-stimulated glucose transport in skeletal muscle is regulated by Rac1. J Physiol. 2015 Feb;593(3):645–56. https://doi.org/10.1113/jphysiol.2014.284281 PMID:25416624
14. Jensen TE, Sylow L, Rose AJ, Madsen AB, Angin Y, Maarbjerg SJ, et al. Contraction-stimulated glucose transport in muscle is controlled by AMPK and mechanical stress but not sarcoplasmatic reticulum Ca(2+) release. Mol Metab. 2014 Jul;3(7):742–53. https://doi.org/10.1016/j.molmet.2014.07.005 PMID:25353002
15. Lackner R, Challiss RA, West D, Newsholme EA. A problem in the radiochemical assay of glucose-6-phosphatase in muscle. Biochem J. 1984 Mar;218(2):649–51. https://doi.org/10.1042/bj2180649 PMID:6324762
16. Virkamäki A, Rissanen E, Hämäläinen S, Utriainen T, Yki-Järvinen H. Incorporation of [3-3H]glucose and 2-[1-14C]deoxyglucose into glycogen in heart and skeletal muscle in vivo: implications for the quantitation of tissue glucose uptake. Diabetes. 1997 Jul;46(7):1106–10. https://doi.org/10.2337/diab.46.7.1106 PMID:9200643
17. Sokoloff L, Reivich M, Kennedy C, Des Rosiers MH, Patlak CS, Pettigrew KD, et al. The [14C]deoxyglucose method for the measurement of local cerebral glucose utilization: theory, procedure, and normal values in the conscious and anesthetized albino rat. J Neurochem. 1977 May;28(5):897–916. https://doi.org/10.1111/j.1471-4159.1977.tb10649.x PMID:864466
18. Ferré P, Leturque A, Burnol AF, Penicaud L, Girard J. A method to quantify glucose utilization in vivo in skeletal muscle and white adipose tissue of the anaesthetized rat. Biochem J. 1985 May;228(1):103–10. https://doi.org/10.1042/bj2280103 PMID:3890836
19. Kipnis DM, Cori CF. Studies of tissue permeability. V. The penetration and phosphorylation of 2-deoxyglucose in the rat diaphragm. J Biol Chem. 1959 Jan;234(1):171–7. https://doi.org/10.1016/S0021-9258(18)70358-X PMID:13610915
20. Forsythe ID, Howells S, Barrett KE. Reproducibility and data presentation. J Physiol. 2019 Nov;597(22):5313. https://doi.org/10.1113/JP277519 PMID:31657457