Lipidomics and temporal-spatial distribution of organelle lipid
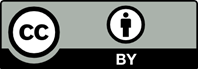
Background: Lipids are crucial signaling molecules or cellular membrane components orchestrating biological processes. To gain insights into lipid functions and the communication between organelles, it is essential to understand the subcellular localization of individual lipids. Advancements in lipid quantification techniques, improvements in chemical and spatial resolution for detecting various lipid species, and enhancements in organelle isolation speed have allowed for profiling of the organelle lipidome, capturing its temporal-spatial distribution. Objective: This review examined approaches used to develop organelle lipidome and aimed to gain insights into cellular lipid homeostasis from an organelle perspective. In addition, this review discussed the advancements in lipid-mediated inter-organelle communication within complex physiological and pathological processes. Conclusion: With the advancement of lipidomic technologies, more detailed explorations of organelle structures and the specific lipid-mediating functions they perform are feasible.
- Jiao HF, Jiang D, Hu XY, et al. Mitocytosis, a migrasome-mediated mitochondrial quality-control process. Cell. 2021;184(11):2896-2910.e2813. doi: 10.1016/j.cell.2021.04.027
- Novikoff AB. Electron microscopy: Cytology of cell fractions. Science. 1956;124(3229):969-972. doi: 10.1126/science.124.3229.969
- Johnstone RM, Bianchini A, Teng K. Reticulocyte maturation and exosome release: Transferrin receptor containing exosomes shows multiple plasma membrane functions. Blood. 1989;74(5):1844-1851. doi: 10.1182/blood.V74.5.1844.1844
- Andreyev AY, Fahy E, Guan Z, et al. Subcellular organelle lipidomics in TLR-4-activated macrophages. J Lipid Res. 2010;51(9):2785-2797. doi: 10.1194/jlr.M008748
- Yao HY, Wang GL, Wang XM. Nuclear translocation ofproteins and the effect of phosphatidic acid. Plant Signal Behav. 2014;9(12):e977711. doi: 10.4161/15592324.2014.977711
- Sakane F, Hoshino F, Murakami C. New era of diacylglycerol kinase, phosphatidic acid and phosphatidic acid-binding protein. Int J Mol Sci. 2020;21(18):6794. doi: 10.3390/ijms21186794
- Yao HY, Xue HW. Phosphatidic acid plays key roles regulating plant development and stress responses. J Integr Plant Biol. 2018;60(9):851-863. doi: 10.1111/jipb.12655
- Nakamura Y, Andrés F, Kanehara K, Liu YC, Dörmann P, Coupland G. Arabidopsis florigen FT binds to diurnally oscillating phospholipids that accelerate flowering. Nat Commun. 2014;5(1):3553. doi: 10.1038/ncomms4553
- Fang Y, Vilella-Bach M, Bachmann R, Flanigan A, Chen J. Phosphatidic acid-mediated mitogenic activation of mTOR signaling. Science. 2001;294(5548):1942-1945. doi: 10.1126/science.1066015
- Watanabe T, Huang HB, Horiuchi A, et al. Protein phosphatase 1 regulation by inhibitors and targeting subunits. Proc Natl Acad Sci U S A. 2001;98(6):3080-3085. doi: 10.1073/pnas.051003898
- Fawcett VCJ, Lorenz U. Localization of Src homology 2 domain-containing phosphatase 1 (SHP-1) to lipid rafts in T lymphocytes: Functional Implications and a Role for the SHP- 1 Carboxyl Terminus1. J Immunol. 2005;174(5):2849-2859. doi: 10.4049/jimmunol.174.5.2849
- Liu XY, Zheng XFS. Endoplasmic reticulum and golgi localization sequences for mammalian target of rapamycin. Mol Biol Cell. 2007;18(3):1073-1082. doi: 10.1091/mbc.e06-05-0406
- Garner K, Hunt AN, Koster G, et al. Phosphatidylinositol transfer protein, cytoplasmic 1 (PITPNC1) binds and transfers phosphatidic acid. J Biol Chem. 2012;287(38):32263-32276. doi: 10.1074/jbc.M112.375840
- Kim YJ, Guzman-Hernandez ML, Wisniewski E, Balla T. Phosphatidylinositol-phosphatidic acid exchange by Nir2 at ER-PM contact sites maintains phosphoinositide signaling competence. Dev Cell. 2015;33(5):549-561. doi: 10.1016/j.devcel.2015.04.028
- Bankaitis VA, Mousley CJ, Schaaf G. The Sec14 superfamily and mechanisms for crosstalk between lipid metabolism and lipid signaling. Trends Biochem Sci. 2010;35(3):150-160. doi: 10.1016/j.tibs.2009.10.008
- Lee RG, Rudler DL, Rackham O, Filipovska A. Interorganelle phospholipid communication, a house not so divided. Trends Endocrinol Metab. 2024;S1043-2760(24):00168-00161. doi: 10.1016/j.tem.2024.06.008
- Lee RG, Rudler DL, Raven SA, et al. Quantitative subcellular reconstruction reveals a lipid mediated inter-organelle biogenesis network. Nat Cell Biol. 2023;26(1):57-71. doi: 10.1038/s41556-023-01297-4
- Wilson JF. Long-suffering lipids gain respect. Scientist. 2003;17:34-36.
- Han XL, Gross RW. Global analyses of cellular lipidomes directly from crude extracts of biological samples by ESI mass spectrometry: A bridge to lipidomics. J Lipid Res. 2003;44(6):1071-1079. doi: 10.1194/jlr.R300004-JLR200
- Spener F, Lagarde M, Géloên A, Record M. Editorial: What is lipidomics? Biochim Biophys Acta. 2003;105(9):481-482. doi: 10.1002/ejlt.200390101
- Klose C, Surma MA, Simons K. Organellar lipidomics - background and perspectives. Curr Opin Cell Biol. 2013;25(4):406-413. doi: 10.1016/j.ceb.2013.03.005
- Lawson EL, Clifton JG, Huang F, Li X, Hixson DC, Josic D. Use of magnetic beads with immobilized monoclonal antibodies for isolation of highly pure plasma membranes. Electrophoresis. 2006;27(13):2747-2758. doi: 10.1002/elps.200600059
- Chang PS, Absood A, Linderman JJ, Omann GM. Magnetic bead isolation of neutrophil plasma membranes and quantification of membrane-associated guanine nucleotide binding proteins. Anal Biochem. 2004;325(2):175-184. doi: 10.1016/j.ab.2003.10.039
- Wang YH, Taylor TH, Arriaga EA. Analysis of the bioactivity of magnetically immunoisolated peroxisomes. Anal Bioanal Chem. 2012;402(1):41-49. doi: 10.1007/s00216-011-5476-3
- Saarela D, Lis P, Gomes S, Nirujogi RS, Dong W, Rawat E, et al. Tagless LysoIP method for molecular profiling of lysosomal content in clinical samples. bioRxiv. 2024;05.17.594681. doi: 10.1101/2024.05.17.594681
- Nylandsted J, Becker AC, Bunkenborg J, Andersen JS, Dengjel J, Jäättelä M. ErbB2-associated changes in the lysosomal proteome. Proteomics. 2011;11(14):2830-2838. doi: 10.1002/pmic.201000734
- Franko A, Baris OR, Bergschneider E, et al. Efficient isolation of pure and functional mitochondria from mouse tissues using automated tissue disruption and enrichment with anti-TOM22 magnetic beads. PLoS One. 2013;8(12):e82392. doi: 10.1371/journal.pone.0082392
- Hornig-Do HT, Günther G, Bust M, Lehnartz P, Bosio A, Wiesner RJ. Isolation of functional pure mitochondria by superparamagnetic microbeads. Anal Biochem. 2009;389(1):1-5. doi: 10.1016/j.ab.2009.02.040
- Guo XN, Hu F, Zhao SH, Yong Z, Zhang ZM, Peng NC. Immunomagnetic separation method integrated with the strep-tag II system for rapid enrichment and mild release of exosomes. Anal Chem. 2023;95(7):3569-3576. doi: 10.1021/acs.analchem.2c03470
- Chen WW, Freinkman E, Wang T, Birsoy K, Sabatini DM. Absolute quantification of matrix metabolites reveals the dynamics of mitochondrial metabolism. Cell. 2016;166(5):1324-1337.e1311. doi: 10.1016/j.cell.2016.07.040
- Chen WW, Freinkman E, Sabatini DM. Rapid immunopurification of mitochondria for metabolite profiling and absolute quantification of matrix metabolites. Nat Protoc. 2017;12(10):2215-2231.doi: 10.1038/nprot.2017.104
- Abu-Remaileh M, Wyant GA, Kim C, et al. Lysosomal metabolomics reveals V-ATPase- and mTOR-dependent regulation of amino acid efflux from lysosomes. Science. 2017;358(6364):807-813. doi: 10.1126/science.aan6298
- Fasimoye R, Dong W, Nirujogi RS, et al. Golgi-IP, a tool for multimodal analysis of Golgi molecular content. Proc Natl Acad Sci U S A. 2023;120(20):e2219953120. doi: 10.1073/pnas.2219953120
- Ray GJ, Boydston EA, Shortt E, et al. A PEROXO-tag enables rapid isolation of peroxisomes from human cells. iScience. 2020;23(5):101109. doi: 10.1016/j.isci.2020.101109
- Xiong J, He J, Xie WP, et al. Rapid affinity purification of intracellular organelles using a twin strep tag. J Cell Sci. 2019;132(24):jcs235390. doi: 10.1242/jcs.235390
- Klemm RW, Ejsing CS, Surma MA, et al. Segregation of sphingolipids and sterols during formation of secretory vesicles at the trans-Golgi network. J Cell Biol. 2009;185(4):601-612. doi: 10.1083/jcb.200901145
- Bayraktar EC, Baudrier L, Ozerdem C, et al. MITO-Tag Mice enable rapid isolation and multimodal profiling of mitochondria from specific cell types in vivo. Proc Natl Acad Sci U S A. 2019;116(1):303-312. doi: 10.1073/pnas.1816656115
- De Mello NP, Fecher C, Pastor AM, Perocchi F, Misgeld T. Ex vivo immunocapture and functional characterization of cell-type-specific mitochondria using MitoTag mice. Nat Protoc. 2023;18(7):2181-2220. doi: 10.1038/s41596-023-00831-w
- Fecher C, Trovò L, Müller SA, et al. Cell-type-specific profiling of brain mitochondria reveals functional and molecular diversity. Nat Neurosci. 2019;22(10):1731-1742. doi: 10.1038/s41593-019-0479-z
- Reinhard J, Starke L, Klose C, et al. MemPrep, a new technology for isolating organellar membranes provides fingerprints of lipid bilayer stress. EMBO J. 2024;43(8):1653-1685. doi: 10.1038/s44318-024-00063-y
- Maitra M, Nagy C, Chawla A, et al. Extraction of nuclei from archived postmortem tissues for single-nucleus sequencing applications. Nat Protoc. 2021;16(6):2788-2801. doi: 10.1038/s41596-021-00514-4
- Frezza C, Cipolat S, Scorrano L. Organelle isolation: Functional mitochondria from mouse liver, muscle and cultured filroblasts. Nat Protoc. 2007;2(2):287-295. doi: 10.1038/nprot.2006.478
- Suski JM, Lebiedzinska M, Wojtala A, et al. Isolation of plasma membrane-associated membranes from rat liver. Nat Protoc. 2014;9(2):312-322. doi: 10.1038/nprot.2014.016
- Mulvey CM, Breckels LM, Geladaki A, et al. Using hyperLOPIT to perform high-resolution mapping of the spatial proteome. Nat Protoc. 2017;12(6):1110-1135. doi: 10.1038/nprot.2017.026
- Sarmento MJ, Llorente A, Petan T, et al. The expanding organelle lipidomes: Current knowledge and challenges. Cell Mol Life Sci. 2023;80(8):237. doi: 10.1007/s00018-023-04889-3
- Schneiter R, Brügger B, Sandhoff R, et al. Electrospray ionization tandem mass spectrometry (Esi-Ms/Ms) analysis of the lipid molecular species composition of yeast subcellular membranes reveals acyl chain-based sorting/remodeling of distinct molecular species en route to the plasma membrane. J Cell Biol. 1999;146(4):741-754. doi: 10.1083/jcb.146.4.741
- Zhu HY, Li QQ, Liao TP, et al. Metabolomic profiling of single enlarged lysosomes. Nat Methods. 2021;18(7):788-798. doi: 10.1038/s41592-021-01182-8
- Zhao YY, Chen Z, Wu Y, et al. Separating and profiling phosphatidylcholines and triglycerides from single cellular lipid droplet by in-tip solvent microextraction mass spectrometry. Anal Chem. 2019;91(7):4466-4471. doi: 10.1021/acs.analchem.8b05122
- Phelps MS, Sturtevant D, Chapman KD, Verbeck GF. Nanomanipulation-coupled matrix-assisted laser desorption/ ionization-direct organelle mass spectrometry: A technique for the detailed analysis of single organelles. J Am Soc Mass Spectrom. 2016;27(2):187-193. doi: 10.1007/s13361-015-1232-9
- Castro DC, Xie YR, Rubakhin SS, Romanova EV, Sweedler JV. Image-guided MALDI mass spectrometry for high-throughput single-organelle characterization. Nat Methods. 2021;18(10):1233-1238. doi: 10.1038/s41592-021-01277-2
- Pliss A, Kuzmin AN, Lita A, et al. A single-organelle optical omics platform for cell science and biomarker discovery. Anal Chem. 2021;93(23):8281-8290. doi: 10.1021/acs.analchem.1c01131
- Lita A, Kuzmin AN, Pliss A, et al. Toward single-organelle lipidomics in live cells. Anal Chem. 2019;91(17):11380-11387. doi: 10.1021/acs.analchem.9b02663
- Lita A, Pliss A, Kuzmin A, et al. IDH1 mutations induce organelle defects via dysregulated phospholipids. Nat Commun. 2021;12(1):614. doi: 10.1038/s41467-020-20752-6
- Bergner G, Albert CR, Schiller M, et al. Quantitative detection of C-deuterated drugs by CARS microscopy and Raman microspectroscopy. Analyst. 2011;136(18):3686-3693. doi: 10.1039/C0AN00956C
- Lin GT, Ning HX, Banie L, et al. Bone marrow cells stained by azide-conjugated alexa fluors in the absence of an alkyne label. Stem Cells Dev. 2012;21(13):2552-2559. doi: 10.1089/scd.2012.0092
- Haberkant P, Stein F, Höglinger D, et al. Bifunctional sphingosine for cell-based analysis of protein-sphingolipid interactions. ACS Chem Biol. 2016;11(1):222-230. doi: 10.1021/acschembio.5b00810
- Feng SH, Harayama T, Montessuit S, et al. Mitochondria-specific photoactivation to monitor local sphingosine metabolism and function. eLife. 2018;7:e34555. doi: 10.7554/eLife.34555
- Ancajas CF, Alam S, Alves DS, et al. Cellular labeling of phosphatidylserine using clickable serine probes. ACS Chem Biol. 2023;18(2):377-384. doi: 10.1021/acschembio.2c00813
- Tamura T, Fujisawa A, Tsuchiya M, et al. Organelle membrane-specific chemical labeling and dynamic imaging in living cells. Nat Chem Biol. 2020;16(12):1361-1367. doi: 10.1038/s41589-020-00651-z
- Rühling M, Kersting L, Wagner F, et al. Trifunctional sphingomyelin derivatives enable nanoscale resolution of sphingomyelin turnover in physiological and infection processes via expansion microscopy. Nat Commun. 2024;15(1):7456. doi: 10.1038/s41467-024-51874-w
- Götz R, Kunz TC, Fink J, et al. Nanoscale imaging of bacterial infections by sphingolipid expansion microscopy. Nat Commun. 2020;11(1):6173. doi: 10.1038/s41467-020-19897-1
- John Peter AT, Petrungaro C, Peter M, Kornmann B. METALIC reveals interorganelle lipid flux in live cells by enzymatic mass tagging. Nat Cell Biol. 2022;24(6):996-1004. doi: 10.1038/s41556-022-00917-9
- Ardail D, Maalouf M, Boivin A, et al. Diversity and complexity of ceramide generation after exposure of jurkat leukemia cells to irradiation. Int J Radiat Oncol Biol Phys. 2009;73(4):1211-1218. doi: 10.1016/j.ijrobp.2008.11.033
- Luo J, Jiang LY, Yang H, Song BL. Intracellular cholesterol transport by sterol transfer proteins at membrane contact sites. Trends Biochem Sci. 2019;44(3):273-292. doi: 10.1016/j.tibs.2018.10.001
- Lee AG. How lipids affect the activities of integral membrane proteins. Biochim Biophys Acta. 2004;1666(1):62-87. doi: 10.1016/j.bbamem.2004.05.012
- Surma MA, Klose C, Klemm RW, Ejsing CS, Simons K. Generic sorting of raft lipids into secretory vesicles in yeast. Traffic. 2011;12(9):1139-1147. doi: 10.1111/j.1600-0854.2011.01221.x
- Bigay J, Antonny B. Curvature, lipid packing, and electrostatics of membrane organelles: Defining cellular territories in determining specificity. Dev Cell. 2012;23(5):886-895. doi: 10.1016/j.devcel.2012.10.009
- Ikonen E. Mechanisms of cellular cholesterol compartmentalization: Recent insights. Curr Opin Cell Biol. 2018;53:77-83. doi: 10.1016/j.ceb.2018.06.002
- Venugopal S, Galano M, Chan R, et al. Dynamic remodeling of membranes and their lipids during acute hormone-induced steroidogenesis in MA-10 mouse leydig tumor cells. Int J Mol Sci. 2021;22(5):2554. doi: 10.3390/ijms22052554
- Lyu K, Zhang Y, Zhang D, et al. A membrane-bound diacylglycerol species induces PKCϵ-mediated hepatic insulin resistance. Cell Metab. 2020;32(4):654-664.e655. doi: 10.1016/j.cmet.2020.08.001
- Lyu K, Zhang D, Song JD, et al. Short-term overnutrition induces white adipose tissue insulin resistance through sn-1,2-diacylglycerol – PKCε – insulin receptorT1160 phosphorylation. JCI Insight. 2021;6(4):e139946. doi: 10.1172/jci.insight.139946
- Gaspar RC, Lyu K, Hubbard BT, et al. Distinct subcellular localisation of intramyocellular lipids and reduced PKCε/ PKCθ activity preserve muscle insulin sensitivity in exercise-trained mice. Diabetologia. 2022;66(3):567-578. doi: 10.1007/s00125-022-05838-8
- Fairn GD, Schieber NL, Ariotti N, et al. High-resolution mapping reveals topologically distinct cellular pools of phosphatidylserine. J Cell Biol. 2011;194(2):257-275. doi: 10.1083/jcb.201012028
- Allan D. Mapping the lipid distribution in the membranes of BHK cells (Mini-Review). Mol Membr Biol. 1996;13(2):81-84. doi: 10.3109/09687689609160580
- Cooke M, Kazanietz MG. Overarching roles of diacylglycerol signaling in cancer development and antitumor immunity. Sci Signal. 2022;15(729):eabo0264. doi: 10.1126/scisignal.abo0264
- Fazio A, Owusu Obeng E, Rusciano I, et al. Subcellular localization relevance and cancer-associated mechanisms of diacylglycerol kinases. Int J Mol Sci. 2020;21(15):5297. doi: 10.3390/ijms21155297
- Joensuu M, Wallis TP, Saber SH, Meunier FA. Phospholipases in neuronal function: A role in learning and memory? J Neurochem. 2020;153(3):300-333. doi: 10.1111/jnc.14918
- Kolczynska K, Loza-Valdes A, Hawro I, Sumara G. Diacylglycerol-evoked activation of PKC and PKD isoforms in regulation of glucose and lipid metabolism: A review. Lipids Health Dis. 2020;19(1):113. doi: 10.1186/s12944-020-01286-8
- Abulizi A, Vatner DF, Ye Z, et al. Membrane-bound sn-1,2- diacylglycerols explain the dissociation of hepatic insulin resistance from hepatic steatosis in MTTP knockout mice. J Lipid Res. 2020;61(12):1565-1576. doi: 10.1194/jlr.RA119000586
- Zheng ZG, Xu YY, Liu WP, et al. Discovery of a potent allosteric activator of DGKQ that ameliorates obesity-induced insulin resistance via the sn-1,2-DAG-PKCε signaling axis. Cell Metab. 2023;35(1):101-117.e111. doi: 10.1016/j.cmet.2022.11.012
- Vance JE. Phospholipid synthesis and transport in mammalian cells. Traffic. 2015;16(1):1-18. doi: 10.1111/tra.12230
- Wenk MR, De Camilli P. Protein-lipid interactions and phosphoinositide metabolism in membrane traffic: Insights from vesicle recycling in nerve terminals. Proc Natl Acad Sci U S A. 2004;101(22):8262-8269. doi: 10.1073/pnas.0401874101
- Di Paolo G, De Camilli P. Phosphoinositides in cell regulation and membrane dynamics. Nature. 2006;443(7112):651-657. doi: 10.1038/nature05185
- De Craene JO, Bertazzi DL, Bar S, Friant S. Phosphoinositides, major actors in membrane trafficking and lipid signaling pathways. Int J Mol Sci. 2017;18(3):634. doi: 10.3390/ijms18030634
- Dickson EJ, Hille B. Understanding phosphoinositides: Rare, dynamic, and essential membrane phospholipids. Biochem J. 2019;476(1):1-23. doi: 10.1042/bcj20180022
- Boal F, Mansour R, Gayral M, et al. TOM1 is a PI5P effector involved in the regulation of endosomal maturation. J Cell Sci. 2015;128(4):815-827. doi: 10.1242/jcs.166314
- Volpatti JR, Al-Maawali A, Smith L, Al-Hashim A, Brill JA, Dowling JJ. The expanding spectrum of neurological disorders of phosphoinositide metabolism. Dis Model Mech. 2019;12(8):dmm038174. doi: 10.1242/dmm.038174
- Fahy E, Subramaniam S, Brown HA, et al. A comprehensive classification system for lipids. J Lipid Res. 2005;46(5):839-861. doi: 10.1194/jlr.E400004-JLR200
- Kuo A, Hla T. Regulation of cellular and systemic sphingolipid homeostasis. Nat Rev Mol Cell Biol. 2024;25(10):802-821. doi: 10.1038/s41580-024-00742-y
- Skotland T, Sandvig K. The role of PS 18:0/18:1 in membrane function. Nat Commun. 2019;10(1):2752. doi: 10.1038/s41467-019-10711-1
- Aviram R, Manella G, Kopelman N, et al. Lipidomics analyses reveal temporal and spatial lipid organization and uncover daily oscillations in intracellular organelles. Mol Cell. 2016;62(4):636-648. doi: 10.1016/j.molcel.2016.04.002
- Vincent AE, White K, Davey T, et al. Quantitative 3D mapping of the human skeletal muscle mitochondrial network. Cell Rep. 2019;27(1):321. doi: 10.1016/j.celrep.2019.03.051
- Zimmermann JA, Lucht K, Stecher M, et al. Functional multi-organelle units control inflammatory lipid metabolism of macrophages. Nat Cell Biol. 2024;26:1261-1273. doi: 10.1038/s41556-024-01457-0
- Domingues N, Pires J, Milosevic I, Raimundo N. Role of lipids in interorganelle communication. Trends Cell Biol. 2024. doi: 10.1016/j.tcb.2024.04.008
- Stefan CJ, Trimble WS, Grinstein S, et al. Membrane dynamics and organelle biogenesis-lipid pipelines and vesicular carriers. BMC Biol. 2017;15(1):102. doi: 10.1186/s12915-017-0432-0
- Li BR, Qin Y, Yu XJ, Xu XW, Yu WY. Lipid raft involvement in signal transduction in cancer cell survival, cell death and metastasis. Cell Prolif. 2022;55(1):e13167. doi: 10.1111/cpr.13167
- Edmond V, Dufour F, Poiroux G, et al. Downregulation of ceramide synthase-6 during epithelial-to-mesenchymal transition reduces plasma membrane fluidity and cancer cell motility. Oncogene. 2015;34(8):996-1005. doi: 10.1038/onc.2014.55
- Liu YY, Hill RA, Li YT. Ceramide glycosylation catalyzed by glucosylceramide synthase and cancer drug resistance. Adv Cancer Res. 2013;117:59-89. doi: 10.1016/B978-0-12-394274-6.00003-0
- Kundu M, Mahata B, Banerjee A, et al. Ganglioside GM2 mediates migration of tumor cells by interacting with integrin and modulating the downstream signaling pathway. Biochim Biophys Acta. 2016;1863(7 Pt A):1472-1489. doi: 10.1016/j.bbamcr.2016.04.004
- Pinho SS, Reis CA. Glycosylation in cancer: Mechanisms and clinical implications. Nat Rev Cancer. 2015;15(9):540-555. doi: 10.1038/nrc3982
- Guo YX, Ma YJ, Han L, Wang YJ, Han JA, Zhu Y. Role of sphingosine 1-phosphate in human pancreatic cancer cells proliferation and migration. Int J Clin Exp Med. 2015;8(11):20349-20354.
- Safaei R, Larson BJ, Cheng TC, et al. Abnormal lysosomal trafficking and enhanced exosomal export of cisplatin in drug-resistant human ovarian carcinoma cells. Mol Cancer Ther. 2005;4(10):1595-1604. doi: 10.1158/1535-7163.Mct-05-0102
- Mashouri L, Yousefi H, Aref AR, Ahadi AM, Molaei F, Alahari SK. Exosomes: Composition, biogenesis, and mechanisms in cancer metastasis and drug resistance. Mol Cancer. 2019;18(1):75. doi: 10.1186/s12943-019-0991-5
- Jafari A, Babajani A, Abdollahpour-Alitappeh M, Ahmadi N, Rezaei-Tavirani M. Exosomes and cancer: From molecular mechanisms to clinical applications. Med Oncol. 2021;38(4):45. doi: 10.1007/s12032-021-01491-0
- Santos NL, Bustos SO, Bhatt D, Chammas R, Andrade LNS. Tumor-derived extracellular vesicles: Modulation of cellular functional dynamics in tumor microenvironment and its clinical implications. Front Cell Dev Biol. 2021;9:737449. doi: 10.3389/fcell.2021.737449
- Moro K, Nagahashi M, Gabriel E, Takabe K, Wakai T. Clinical application of ceramide in cancer treatment. Breast Cancer. 2019;26(4):407-415. doi: 10.1007/s12282-019-00953-8
- Don AS, Lim XY, Couttas TA. Re-configuration of sphingolipid metabolism by oncogenic transformation. Biomolecules. 2014;4(1):315-353. doi: 10.3390/biom4010315
- Li M, Li H, Yang S, Liao X, Zhao C, Wang F. L-carnitine attenuates TGF-β1-induced EMT in retinal pigment epithelial cells via a PPARγ-dependent mechanism. Int J Mol Med. 2021;47(6):110. doi: 10.10.3892/ijmm.2021.4943
- Rahajeng J, Kuna RS, Makowski SL, et al. Efficient Golgi forward trafficking requires GOLPH3-Driven, PI4P-dependent membrane curvature. Dev Cell. 2019;50(5):573-585.e575. doi: 10.1016/j.devcel.2019.05.038
- Kuna RS, Field SJ. GOLPH3: A Golgi phosphatidylinositol(4) phosphate effector that directs vesicle trafficking and drives cancer. J Lipid Res. 2019;60(2):269-275. doi: 10.1194/jlr.R088328
- Xing M, Peterman MC, Davis RL, Oegema K, Shiau AK, Field SJ. GOLPH3 drives cell migration by promoting Golgi reorientation and directional trafficking to the leading edge. Mol Biol Cell. 2016;27(24):3828-3840. doi: 10.1091/mbc.E16-01-0005
- Anitei M, Stange C, Czupalla C, et al. Spatiotemporal control of lipid conversion, actin-based mechanical forces, and curvature sensors during Clathrin/AP-1-coated vesicle biogenesis. Cell Rep. 2017;20(9):2087-2099. doi: 10.1016/j.celrep.2017.08.013
- Irvine RF. Nuclear lipid signalling. Nat Rev Mol Cell Biol. 2003;4(5):349-360. doi: 10.1038/nrm1100
- Dahabieh MS, Di Pietro E, Jangal M, et al. Peroxisomes and cancer: The role of a metabolic specialist in a disease of aberrant metabolism. Biochim Biophys Acta. 2018;1870(1):103-121. doi: 10.1016/j.bbcan.2018.07.004
- Ballabio A, Bonifacino JS. Lysosomes as dynamic regulators of cell and organismal homeostasis. Nat Rev Mol Cell Biol. 2020;21(2):101-118. doi: 10.1038/s41580-019-0185-4
- Cui YF, Man SL, Tao JJ, et al. The lipid droplet in cancer: From being a tumor-supporting hallmark to clinical therapy. Acta Physiol (Oxf). 2024;240(3):e14087. doi: 10.1111/apha.14087
- Fu Y, Zou TT, Shen XT, et al. Lipid metabolism in cancer progression and therapeutic strategies. MedComm. 2021;2(1):27-59. doi: 10.1002/mco2.27
- Jeong DW, Lee S, Chun YS. How cancer cells remodel lipid metabolism: Strategies targeting transcription factors. Lipids Health Dis. 2021;20(1):163. doi: 10.1186/s12944-021-01593-8
- Hu JM, Zhang LY, Chen WZ, et al. Role of intra- and extracellular lipid signals in cancer stemness and potential therapeutic strategy. Front Pharmacol. 2021;12:730751. doi: 10.3389/fphar.2021.730751