Comparison of CRISPR and adenovirus-mediated Myd88 knockdown in RAW 264.7 cells and responses to lipopolysaccharide stimulation
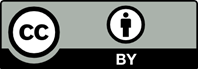
Genomic manipulation offers the possibility for novel therapies in lieu of medical interventions in use today. The ability to
genetically restore missing inflammatory genes will have a monumental impact on our current immunotherapy treatments. This study compared the efficacy of two different genetic manipulation techniques: clustered regularly interspaced short palindromic repeats (CRISPR)/CRISPR associated protein 9 (Cas9) transfection to adenoviral transduction to determine which method would provide the most transient and stable knockdown of myeloid differentiation primary response 88 (MyD88). MyD88 is a major regulator of nuclear factor kappa light chain enhancer of activated B cells (NFκB) pathway in Raw 264.7 macrophages. Following genetic manipulation, cells were treated for 24 h with Lipopolysaccharide (LPS) to stimulate the inflammatory pathway. Confirmation of knockdown was determined by western immunoblotting and quantification of band density. Both CRISPR/Cas9 and adenoviral transduction produced similar knockdown efficiency (~64% and 60%, respectively) in MyD88 protein 48 h post adenoviral transduction. NFκB phosphorylation was increased in CRISPR/Cas9-mediated MyD88 knockdown and control cells, but not in adenovirus-mediated MyD88 knockdown cells, following LPS administration. CRISPR/Cas9-mediated MyD88 knockdown macrophages treated with LPS for 24 h showed a 65% reduction in tumor necrosis factor alpha (TNFα) secretion, and a 67% reduction in interleukin-10 (IL-10) secretion when compared to LPS-stimulated control cells (P ≤ 0.01 for both). LPS did not stimulate TNFα or IL-10 secretion in adenovirus-mediated control or MyD88 knockdown cells. These data demonstrate that Raw 264.7 macrophages maintain responsiveness to inflammatory stimuli following CRISPR/Cas9-mediated reductions in MyD88, but not following adenovirus-mediated MyD88 knockdown.
1. Hoyt JC, Robbins RA. Macrolide antibiotics and pulmonary inflammation. FEMS Microbiol Lett. 2001 Nov;205(1):1–7. https://doi.org/10.1111/j.1574-6968.2001.tb10917.x PMID:11728708
2. Melero-Jerez C, Ortega MC, Moliné-Velázquez V, Clemente D. Myeloid derived suppressor cells in inflammatory conditions of the central nervous system. Biochim Biophys Acta. 2016 Mar;1862(3):368–80. https://doi.org/10.1016/j.bbadis.2015.10.015 PMID:26527182
3. Yi Y, Noh MJ, Lee KH. Current advances in retroviral gene therapy. Curr Gene Ther. 2011 Jun;11(3):218–28. https://doi.org/10.2174/156652311795684740 PMID:21453283
4. Zhou Y, Boudreau DM, Freedman AN. Trends in the use of aspirin and nonsteroidal anti-inflammatory drugs in the general U.S. population. Pharmacoepidemiol Drug Saf. 2014 Jan;23(1):43–50. https://doi.org/10.1002/pds.3463 PMID:23723142
5. Coxib, traditional NTC, Bhala N, Emberson J, Merhi A, Abramson S, et al. Vascular and upper gastrointestinal effects of non-steroidal anti-inflammatory drugs: meta-analyses of individual participant data from randomised trials. Lancet. 2013;382(9894):769-79.
6. Lang FF, Bruner JM, Fuller GN, Aldape K, Prados MD, Chang S, et al. Phase I trial of adenovirus-mediated p53 gene therapy for recurrent glioma: biological and clinical results. J Clin Oncol. 2003 Jul;21(13):2508–18. https://doi.org/10.1200/JCO.2003.21.13.2508 PMID:12839017
7. Sakurai F, Mizuguchi H, Yamaguchi T, Hayakawa T. Characterization of in vitro and in vivo gene transfer properties of adenovirus serotype 35 vector. Mol Ther. 2003 Nov;8(5):813–21. https://doi.org/10.1016/S1525-0016(03)00243-0 PMID:14599815
8. Wang H, La Russa M, Qi LS. CRISPR/Cas9 in Genome Editing and Beyond. Annu Rev Biochem. 2016 Jun;85(1):227–64. https://doi.org/10.1146/annurev-biochem-060815-014607 PMID:27145843
9. Yeh P, Perricaudet M. Advances in adenoviral vectors: from genetic engineering to their biology. FASEB J. 1997 Jul;11(8):615–23. https://doi.org/10.1096/fasebj.11.8.9240963 PMID:9240963
10. Ohashi M, Kanai F, Ueno H, Tanaka T, Tateishi K, Kawakami T, et al. Adenovirus mediated p53 tumour suppressor gene therapy for human gastric cancer cells in vitro and in vivo. Gut. 1999 Mar;44(3):366–71. https://doi.org/10.1136/gut.44.3.366 PMID:10026322
11. Ryuke Y, Mizuno M, Natsume A, Yoshida J. Transduction efficiency of adenoviral vectors into human glioma cells increased by association with cationic liposomes. Neurol Med Chir (Tokyo). 2000 May;40(5):256–60. https://doi.org/10.2176/nmc.40.256 PMID:11980090
12. Liao HK, Gu Y, Diaz A, Marlett J, Takahashi Y, Li M, et al. Use of the CRISPR/Cas9 system as an intracellular defense against HIV-1 infection in human cells. Nat Commun. 2015 Mar;6(1):6413. https://doi.org/10.1038/ncomms7413 PMID:25752527
13. Guo R, Wan Y, Xu D, Cui L, Deng M, Zhang G, et al. Generation and evaluation of Myostatin knock-out rabbits and goats using CRISPR/Cas9 system. Sci Rep. 2016 Jul;6(1):29855. https://doi.org/10.1038/srep29855 PMID:27417210
14. Ran FA, Hsu PD, Wright J, Agarwala V, Scott DA, Zhang F. Genome engineering using the CRISPR-Cas9 system. Nat Protoc. 2013 Nov;8(11):2281–308. https://doi.org/10.1038/nprot.2013.143 PMID:24157548
15. Zhang XH, Tee LY, Wang XG, Huang QS, Yang SH. Off-target Effects in CRISPR/Cas9-mediated Genome Engineering. Mol Ther Nucleic Acids. 2015 Nov;4:e264. https://doi.org/10.1038/mtna.2015.37 PMID:26575098
16. Lau CH, Suh Y. In vivo genome editing in animals using AAV-CRISPR system: applications to translational research of human disease. F1000 Res. 2017 Dec;6:2153. https://doi.org/10.12688/f1000research.11243.1 PMID:29333255
17. Dow LE, Fisher J, O’Rourke KP, Muley A, Kastenhuber ER, Livshits G, et al. Inducible in vivo genome editing with CRISPR-Cas9. Nat Biotechnol. 2015 Apr;33(4):390–4. https://doi.org/10.1038/nbt.3155 PMID:25690852
18. Lawrence T. The nuclear factor NF-kappaB pathway in inflammation. Cold Spring Harb Perspect Biol. 2009 Dec;1(6):a001651. https://doi.org/10.1101/cshperspect.a001651 PMID:20457564
19. Wang JQ, Jeelall YS, Ferguson LL, Horikawa K. Toll-Like Receptors and Cancer: MYD88 Mutation and Inflammation. Front Immunol. 2014 Jul;5:367. https://doi.org/10.3389/fimmu.2014.00367 PMID:25132836
20. Hobbs S, Reynoso M, Geddis AV, Mitrophanov AY, Matheny RW Jr. LPS-stimulated NF-κB p65 dynamic response marks the initiation of TNF expression and transition to IL-10 expression in RAW 264.7 macrophages. Physiol Rep. 2018 Nov;6(21):e13914. https://doi.org/10.14814/phy2.13914 PMID:30426723
21. Bradford MM. A rapid and sensitive method for the quantitation of microgram quantities of protein utilizing the principle of protein-dye binding. Anal Biochem. 1976 May;72(1-2):248–54. https://doi.org/10.1016/0003-2697(76)90527-3 PMID:942051
22. Matheny RW Jr, Lynch CM, Leandry LA. Enhanced Akt phosphorylation and myogenic differentiation in PI3K p110β-deficient myoblasts is mediated by PI3K p110α and mTORC2. Growth Factors. 2012 Dec;30(6):367–84. https://doi.org/10.3109/08977194.2012.734507 PMID:23137199
23. Schneider CA, Rasband WS, Eliceiri KW. NIH Image to ImageJ: 25 years of image analysis. Nat Methods. 2012 Jul;9(7):671–5. https://doi.org/10.1038/nmeth.2089 PMID:22930834
24. Yamada R, Ymamoto K. Recent findings on genes associated with inflammatory disease. Mutat Res. 2005 Jun;573(1-2):136–51. https://doi.org/10.1016/j.mrfmmm.2004.06.061 PMID:15829243
25. Tuppen HA, Blakely EL, Turnbull DM, Taylor RW. Mitochondrial DNA mutations and human disease. Biochim Biophys Acta. 2010 Feb;1797(2):113–28. https://doi.org/10.1016/j.bbabio.2009.09.005 PMID:19761752
26. Wilmut I, Hooper ML, Simons JP. Genetic manipulation of mammals and its application in reproductive biology. J Reprod Fertil. 1991 Jul;92(2):245–79. https://doi.org/10.1530/jrf.0.0920245 PMID:1886087
27. van der Weyden L, Adams DJ, Bradley A. Tools for targeted manipulation of the mouse genome. Physiol Genomics. 2002 Dec;11(3):133–64. https://doi.org/10.1152/physiolgenomics.00074.2002 PMID:12464689
28. Corridon PR, Rhodes GJ, Leonard EC, Basile DP, Gattone VH 2nd, Bacallao RL, et al. A method to facilitate and monitor expression of exogenous genes in the rat kidney using plasmid and viral vectors. Am J Physiol Renal Physiol. 2013 May;304(9):F1217–29. https://doi.org/10.1152/ajprenal.00070.2013 PMID:23467422
29. Veach RA, Wilson MH. CRISPR/Cas9 engineering of a KIM-1 reporter human proximal tubule cell line. PLoS One. 2018 Sep;13(9):e0204487. https://doi.org/10.1371/journal.pone.0204487 PMID:30260998
30. Matheny RW Jr, Riddle-Kottke MA, Leandry LA, Lynch CM, Abdalla MN, Geddis AV, et al. Role of phosphoinositide 3-OH kinase p110β in skeletal myogenesis. Mol Cell Biol. 2015 Apr;35(7):1182–96. https://doi.org/10.1128/MCB.00550-14 PMID:25605332