Primary cell-based phenotypic assays to pharmacologically and genetically study fibrotic diseases in vitro
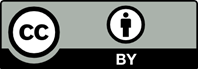
Ongoing tissue repair and formation and deposition of collagen-rich extracellular matrix in tissues and organs finally lead to fibrotic lesions and destruction of normal tissue/organ architecture and function. In the lung, scarring is observed in asthma, chronic obstructive pulmonary disease and idiopathic pulmonary fibrosis to various degrees. At the cellular level immune cells, fibroblasts and epithelial cells are all involved in fibrotic processes. Mechanistically, fibroblast to myofibroblast transformation and epithelial to mesenchymal transition are major drivers of fibrosis. Amongst others, both processes are controlled by transforming growth factor beta-1 (TGFβ-1), a growth factor upregulated in idiopathic pulmonary fibrosis lungs. Phenotypic assays with primary human cells and complex disease-relevant readouts become
increasingly important in modern drug discovery processes. We describe high-content screening based phenotypic assays with primary normal human lung fibroblasts and primary human airway epithelial cells. For both cell types, TGFβ-1 stimulation is used to induce fibrotic phenotypes in vitro, with alpha smooth muscle actin and collagen-I as readouts for FMT and E-cadherin as a readout for EMT. For each assay, a detailed image analysis protocols is described. Treatment of both cell types with TGFβ-1 and a transforming growth factor beta receptor inhibitor verifies the suitability of the assays for pharmacological interventions. In addition, the assays are compatible for siRNA and Cas9-ribonucleoprotein transfections, and thus are useful for genetic target identification/validation by modulating gene expression.
1. Pakshir P, Hinz B. The big five in fibrosis: Macrophages, myofibroblasts, matrix, mechanics, and miscommunication. Matrix Biol. 2018;68-69:81-93. Epub 2018/02/07. doi: 10.1016/j.matbio.2018.01.019. PubMed PMID: 29408013.
2. Weiskirchen R, Weiskirchen S, Tacke F. Organ and tissue fibrosis: Molecular signals, cellular mechanisms and translational implications. Mol Aspects Med. 2018. Epub 2018/07/01. doi: 10.1016/j.mam.2018.06.003. PubMed PMID: 29958900.
3. Yasukawa A, Hosoki K, Toda M, Miyake Y, Matsushima Y, Matsumoto T, et al. Eosinophils promote epithelial to mesenchymal transition of bronchial epithelial cells. PLoS One. 2013;8(5):e64281. Epub 2013/05/24. doi: 10.1371/journal.pone.0064281. PubMed PMID: 23700468; PubMed Central PMCID: PMCPMC3660301.
4. Yang X, Liang L, Zong C, Lai F, Zhu P, Liu Y, et al. Kupffer cells-dependent inflammation in the injured liver increases recruitment of mesenchymal stem cells in aging mice. Oncotarget. 2016;7(2):1084-95. Epub 2015/12/31. doi: 10.18632/oncotarget.6744. PubMed PMID: 26716516; PubMed Central PMCID: PMCPMC4811445.
5. Kalluri R, Neilson EG. Epithelial-mesenchymal transition and its implications for fibrosis. J Clin Invest. 2003;112(12):1776-84. Epub 2003/12/18. doi: 10.1172/JCI20530. PubMed PMID: 14679171; PubMed Central PMCID: PMCPMC297008.
6. Karicheva O, Rodriguez-Vargas JM, Wadier N, Martin-Hernandez K, Vauchelles R, Magroun N, et al. PARP3 controls TGFbeta and ROS driven epithelial-to-mesenchymal transition and stemness by stimulating a TG2-Snail-E-cadherin axis. Oncotarget. 2016;7(39):64109-23. Epub 2016/09/01. doi: 10.18632/oncotarget.11627. PubMed PMID: 27579892; PubMed Central PMCID: PMCPMC5325429.
7. Rout-Pitt N, Farrow N, Parsons D, Donnelley M. Epithelial mesenchymal transition (EMT): a universal process in lung diseases with implications for cystic fibrosis pathophysiology. Respir Res. 2018;19(1):136. Epub 2018/07/20. doi: 10.1186/s12931-018-0834-8. PubMed PMID: 30021582; PubMed Central PMCID: PMCPMC6052671.
8. Johnson JR, Nishioka M, Chakir J, Risse PA, Almaghlouth I, Bazarbashi AN, et al. IL-22 contributes to TGF-beta1-mediated epithelial-mesenchymal transition in asthmatic bronchial epithelial cells. Respir Res. 2013;14:118. Epub 2013/11/29. doi: 10.1186/1465-9921-14-118. PubMed PMID: 24283210; PubMed Central PMCID: PMCPMC4176096.
9. Jonsdottir HR, Arason AJ, Palsson R, Franzdottir SR, Gudbjartsson T, Isaksson HJ, et al. Basal cells of the human airways acquire mesenchymal traits in idiopathic pulmonary fibrosis and in culture. Lab Invest. 2015;95(12):1418-28. Epub 2015/09/22. doi: 10.1038/labinvest.2015.114. PubMed PMID: 26390052.
10. Moffat JG, Vincent F, Lee JA, Eder J, Prunotto M. Opportunities and challenges in phenotypic drug discovery: an industry perspective. Nat Rev Drug Discov. 2017;16(8):531-43. Epub 2017/07/08. doi: 10.1038/nrd.2017.111. PubMed PMID: 28685762.
11. Vincent F, Loria P, Pregel M, Stanton R, Kitching L, Nocka K, et al. Developing predictive assays: the phenotypic screening "rule of 3". Sci Transl Med. 2015;7(293):293ps15. Epub 2015/06/26. doi: 10.1126/scitranslmed.aab1201. PubMed PMID: 26109101.
12. Camara J, Jarai G. Epithelial-mesenchymal transition in primary human bronchial epithelial cells is Smad-dependent and enhanced by fibronectin and TNF-alpha. Fibrogenesis Tissue Repair. 2010;3(1):2. Epub 2010/01/07. doi: 10.1186/1755-1536-3-2. PubMed PMID: 20051102; PubMed Central PMCID: PMCPMC2821296.
13. Voets O, Tielen F, Elstak E, Benschop J, Grimbergen M, Stallen J, et al. Highly efficient gene inactivation by adenoviral CRISPR/Cas9 in human primary cells. PLoS One. 2017;12(8):e0182974. Epub 2017/08/12. doi: 10.1371/journal.pone.0182974. PubMed PMID: 28800587; PubMed Central PMCID: PMCPMC5553774.
14. Zhao L, Xu Y, Tao L, Yang Y, Shen X, Li L, et al. Oxymatrine Inhibits Transforming Growth Factor beta1 (TGF-beta1)-Induced Cardiac Fibroblast-to-Myofibroblast Transformation (FMT) by Mediating the Notch Signaling Pathway In Vitro. Med Sci Monit. 2018;24:6280-8. Epub 2018/09/10. doi: 10.12659/MSM.910142. PubMed PMID: 30196308; PubMed Central PMCID: PMCPMC6142867.
15. Jung H, Lee DS, Park SK, Choi JS, Jung WK, Park WS, et al. Fucoxanthin Inhibits Myofibroblast Differentiation and Extracellular Matrix Production in Nasal Polyp-Derived Fibroblasts via Modulation of Smad-Dependent and Smad-Independent Signaling Pathways. Mar Drugs. 2018;16(9). Epub 2018/09/12. doi: 10.3390/md16090323. PubMed PMID: 30201895; PubMed Central PMCID: PMCPMC6165344.
16. Qu MH, Han C, Srivastava AK, Cui T, Zou N, Gao ZQ, et al. miR-93 promotes TGF-beta-induced epithelial-to-mesenchymal transition through downregulation of NEDD4L in lung cancer cells. Tumour Biol. 2016;37(4):5645-51. Epub 2015/11/20. doi: 10.1007/s13277-015-4328-8. PubMed PMID: 26581907.
17. Toba-Ichihashi Y, Yamaoka T, Ohmori T, Ohba M. Up-regulation of Syndecan-4 contributes to TGF-beta1-induced epithelial to mesenchymal transition in lung adenocarcinoma A549 cells. Biochem Biophys Rep. 2016;5:1-7. Epub 2015/11/27. doi: 10.1016/j.bbrep.2015.11.021. PubMed PMID: 28955801; PubMed Central PMCID: PMCPMC5600357.
18. Khalil N, O'Connor RN, Flanders KC, Unruh H. TGF-beta 1, but not TGF-beta 2 or TGF-beta 3, is differentially present in epithelial cells of advanced pulmonary fibrosis: an immunohistochemical study. Am J Respir Cell Mol Biol. 1996;14(2):131-8. Epub 1996/02/01. doi: 10.1165/ajrcmb.14.2.8630262. PubMed PMID: 8630262.
19. Coker RK, Laurent GJ, Shahzeidi S, Lympany PA, du Bois RM, Jeffery PK, et al. Transforming growth factors-beta 1, -beta 2, and -beta 3 stimulate fibroblast procollagen production in vitro but are differentially expressed during bleomycin-induced lung fibrosis. Am J Pathol. 1997;150(3):981-91. Epub 1997/03/01. PubMed PMID: 9060836; PubMed Central PMCID: PMCPMC1857875.
20. King TE, Jr., Pardo A, Selman M. Idiopathic pulmonary fibrosis. Lancet. 2011;378(9807):1949-61. Epub 2011/07/02. doi: 10.1016/S0140-6736(11)60052-4. PubMed PMID: 21719092.
21. Selman M, Pardo A. Revealing the pathogenic and aging-related mechanisms of the enigmatic idiopathic pulmonary fibrosis. an integral model. Am J Respir Crit Care Med. 2014;189(10):1161-72. Epub 2014/03/20. doi: 10.1164/rccm.201312-2221PP. PubMed PMID: 24641682.
22. Wuyts WA, Agostini C, Antoniou KM, Bouros D, Chambers RC, Cottin V, et al. The pathogenesis of pulmonary fibrosis: a moving target. Eur Respir J. 2013;41(5):1207-18. Epub 2012/10/27. doi: 10.1183/09031936.00073012. PubMed PMID: 23100500.
23. Mohr SE, Smith JA, Shamu CE, Neumuller RA, Perrimon N. RNAi screening comes of age: improved techniques and complementary approaches. Nat Rev Mol Cell Biol. 2014;15(9):591-600. Epub 2014/08/26. doi: 10.1038/nrm3860. PubMed PMID: 25145850; PubMed Central PMCID: PMCPMC4204798.
24. Kabadi AM, Ousterout DG, Hilton IB, Gersbach CA. Multiplex CRISPR/Cas9-based genome engineering from a single lentiviral vector. Nucleic Acids Res. 2014;42(19):e147. Epub 2014/08/15. doi: 10.1093/nar/gku749. PubMed PMID: 25122746; PubMed Central PMCID: PMCPMC4231726.
25. Bellec J, Bacchetta M, Losa D, Anegon I, Chanson M, Nguyen TH. CFTR inactivation by lentiviral vector-mediated RNA interference and CRISPR-Cas9 genome editing in human airway epithelial cells. Curr Gene Ther. 2015;15(5):447-59. Epub 2015/08/13. PubMed PMID: 26264708.
26. Schuster A, Erasimus H, Fritah S, Nazarov PV, van Dyck E, Niclou SP, et al. RNAi/CRISPR Screens: from a Pool to a Valid Hit. Trends Biotechnol. 2018. Epub 2018/09/05. doi: 10.1016/j.tibtech.2018.08.002. PubMed PMID: 30177380.
27. Kim S, Kim D, Cho SW, Kim J, Kim JS. Highly efficient RNA-guided genome editing in human cells via delivery of purified Cas9 ribonucleoproteins. Genome Res. 2014;24(6):1012-9. Epub 2014/04/04. doi: 10.1101/gr.171322.113. PubMed PMID: 24696461; PubMed Central PMCID: PMCPMC4032847.
28. Liu J, Gaj T, Yang Y, Wang N, Shui S, Kim S, et al. Efficient delivery of nuclease proteins for genome editing in human stem cells and primary cells. Nat Protoc. 2015;10(11):1842-59. Epub 2015/10/23. doi: 10.1038/nprot.2015.117. PubMed PMID: 26492140.
29. Aumiller V, Strobel B, Romeike M, Schuler M, Stierstorfer BE, Kreuz S. Comparative analysis of lysyl oxidase (like) family members in pulmonary fibrosis. Sci Rep. 2017;7(1):149. Epub 2017/03/10. doi: 10.1038/s41598-017-00270-0. PubMed PMID: 28273952; PubMed Central PMCID: PMCPMC5428068.
30. Hsiau T, Maures T, Waite K, Yang J, Kelso R, Holden K, et al. Inference of CRISPR Edits from Sanger Trace Data. bioRxiv. 2018. doi: 10.1101/251082.
31. Ijaz T, Pazdrak K, Kalita M, Konig R, Choudhary S, Tian B, et al. Systems biology approaches to understanding Epithelial Mesenchymal Transition (EMT) in mucosal remodeling and signaling in asthma. World Allergy Organ J. 2014;7(1):13. Epub 2014/07/02. doi: 10.1186/1939-4551-7-13. PubMed PMID: 24982697; PubMed Central PMCID: PMCPMC4068075.
32. Zent J, Guo LW. Signaling Mechanisms of Myofibroblastic Activation: Outside-in and Inside-Out. Cell Physiol Biochem. 2018;49(3):848-68. Epub 2018/09/06. doi: 10.1159/000493217. PubMed PMID: 30184544.
33. Swinney DC, Anthony J. How were new medicines discovered? Nat Rev Drug Discov. 2011;10(7):507-19. Epub 2011/06/28. doi: 10.1038/nrd3480. PubMed PMID: 21701501.
34. Scannell JW, Bosley J. When Quality Beats Quantity: Decision Theory, Drug Discovery, and the Reproducibility Crisis. PLoS One. 2016;11(2):e0147215. Epub 2016/02/11. doi: 10.1371/journal.pone.0147215. PubMed PMID: 26863229; PubMed Central PMCID: PMCPMC4749240.
35. Bollong MJ, Yang B, Vergani N, Beyer BA, Chin EN, Zambaldo C, et al. Small molecule-mediated inhibition of myofibroblast transdifferentiation for the treatment of fibrosis. Proc Natl Acad Sci U S A. 2017;114(18):4679-84. Epub 2017/04/19. doi: 10.1073/pnas.1702750114. PubMed PMID: 28416697; PubMed Central PMCID: PMCPMC5422806.
36. Wang XT, Sun XJ, Li C, Liu Y, Zhang L, Li YD, et al. Establishing a Cell-Based High-Content Screening Assay for TCM Compounds with Anti-Renal Fibrosis Effects. Evid Based Complement Alternat Med. 2018;2018:7942614. Epub 2018/07/28. doi: 10.1155/2018/7942614. PubMed PMID: 30050593; PubMed Central PMCID: PMCPMC6046160.
37. Talele NP, Fradette J, Davies JE, Kapus A, Hinz B. Expression of alpha-Smooth Muscle Actin Determines the Fate of Mesenchymal Stromal Cells. Stem Cell Reports. 2015;4(6):1016-30. Epub 2015/06/02. doi: 10.1016/j.stemcr.2015.05.004. PubMed PMID: 26028530; PubMed Central PMCID: PMCPMC4471834.
38. Oh RS, Haak AJ, Smith KMJ, Ligresti G, Choi KM, Xie T, et al. RNAi screening identifies a mechanosensitive ROCK-JAK2-STAT3 network central to myofibroblast activation. J Cell Sci. 2018;131(10). Epub 2018/04/22. doi: 10.1242/jcs.209932. PubMed PMID: 29678906; PubMed Central PMCID: PMCPMC6031327.
39. Lareu RR, Arsianti I, Subramhanya HK, Yanxian P, Raghunath M. In vitro enhancement of collagen matrix formation and crosslinking for applications in tissue engineering: a preliminary study. Tissue Eng. 2007;13(2):385-91. Epub 2007/05/24. doi: 10.1089/ten.2006.0224. PubMed PMID: 17518571.
40. Lareu RR, Subramhanya KH, Peng Y, Benny P, Chen C, Wang Z, et al. Collagen matrix deposition is dramatically enhanced in vitro when crowded with charged macromolecules: the biological relevance of the excluded volume effect. FEBS Lett. 2007;581(14):2709-14. Epub 2007/05/29. doi: 10.1016/j.febslet.2007.05.020. PubMed PMID: 17531987.
41. Chen CZ, Peng YX, Wang ZB, Fish PV, Kaar JL, Koepsel RR, et al. The Scar-in-a-Jar: studying potential antifibrotic compounds from the epigenetic to extracellular level in a single well. Br J Pharmacol. 2009;158(5):1196-209. Epub 2009/09/30. doi: 10.1111/j.1476-5381.2009.00387.x. PubMed PMID: 19785660; PubMed Central PMCID: PMCPMC2782330.
42. Carthy JM, Stoter M, Bellomo C, Vanlandewijck M, Heldin A, Moren A, et al. Chemical regulators of epithelial plasticity reveal a nuclear receptor pathway controlling myofibroblast differentiation. Sci Rep. 2016;6:29868. Epub 2016/07/20. doi: 10.1038/srep29868. PubMed PMID: 27430378; PubMed Central PMCID: PMCPMC4949434.
43. Lotz-Jenne C, Luthi U, Ackerknecht S, Lehembre F, Fink T, Stritt M, et al. A high-content EMT screen identifies multiple receptor tyrosine kinase inhibitors with activity on TGFbeta receptor. Oncotarget. 2016;7(18):25983-6002. Epub 2016/04/02. doi: 10.18632/oncotarget.8418. PubMed PMID: 27036020; PubMed Central PMCID: PMCPMC5041959.
44. Pavan S, Meyer-Schaller N, Diepenbruck M, Kalathur RKR, Saxena M, Christofori G. A kinome-wide high-content siRNA screen identifies MEK5-ERK5 signaling as critical for breast cancer cell EMT and metastasis. Oncogene. 2018;37(31):4197-213. Epub 2018/05/02. doi: 10.1038/s41388-018-0270-8. PubMed PMID: 29713055.
45. Liang X, Potter J, Kumar S, Zou Y, Quintanilla R, Sridharan M, et al. Rapid and highly efficient mammalian cell engineering via Cas9 protein transfection. J Biotechnol. 2015;208:44-53. Epub 2015/05/25. doi: 10.1016/j.jbiotec.2015.04.024. PubMed PMID: 26003884.
46. Seki A, Rutz S. Optimized RNP transfection for highly efficient CRISPR/Cas9-mediated gene knockout in primary T cells. J Exp Med. 2018;215(3):985-97. Epub 2018/02/14. doi: 10.1084/jem.20171626. PubMed PMID: 29436394; PubMed Central PMCID: PMCPMC5839763.
47. Farboud B, Jarvis E, Roth TL, Shin J, Corn JE, Marson A, et al. Enhanced Genome Editing with Cas9 Ribonucleoprotein in Diverse Cells and Organisms. J Vis Exp. 2018;(135). Epub 2018/06/12. doi: 10.3791/57350. PubMed PMID: 29889198; PubMed Central PMCID: PMCPMC6101420.
48. Dominguez AA, Lim WA, Qi LS. Beyond editing: repurposing CRISPR-Cas9 for precision genome regulation and interrogation. Nat Rev Mol Cell Biol. 2016;17(1):5-15. Epub 2015/12/17. doi: 10.1038/nrm.2015.2. PubMed PMID: 26670017; PubMed Central PMCID: PMCPMC4922510.
49. Michalik M, Pierzchalska M, Wlodarczyk A, Wojcik KA, Czyz J, Sanak M, et al. Transition of asthmatic bronchial fibroblasts to myofibroblasts is inhibited by cell-cell contacts. Respir Med. 2011;105(10):1467-75. Epub 2011/08/02. doi: 10.1016/j.rmed.2011.04.009. PubMed PMID: 21802932.