Silk coating as a novel delivery system and reversible adhesive for stiffening and shaping flexible probes
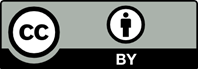
The performance of any implantable electrode depends not only on its recording or stimulation capabilities but also on its position in relation to the target site. Electrode displacement during or after implantation represents a major issue as it might result in tissue damage or incorrect recording or stimulation location, complicating the interpretation of experimental data. Although thin-film electrode arrays have overcome some of the main limitations of more traditional, stiffer probes, their intrinsic flexibility and unilateral contacts represent a new challenge: they tend to bend during insertion and are difficult to implant simultaneously while maintaining a specific relative position. Here, we present a method that addresses all these issues using a coating of silk fibroin, a versatile protein derived from silkworm cocoons. The method is demonstrated by acquiring electromyographic (EMG) recordings in Manduca sexta, a soft-bodied animal that exemplifies the issues of electrode insertion and placement in delicate and deformable tissues.
[1] Voldman J, Gray LG, Schmidt MA (1995) Microfabrication in biology and medicine. Annu Rev Biomed Eng 1: 401–425.
[2] Kipke, D. R., D. S. Pellinen, Vetter RJ (2002) Advanced neural implants using thin-film polymers. IEEE International Symposium on Circuits and Systems (ISCAS) 4: 173-176.
[3] Grill WM, Norman SE, Bellamkonda RV (2009) Implanted neural interfaces: biochallenges and engineered solutions. Annu Rev Biomed Eng 11: 1-24.
[4] Babb MI, Dymond AM (1975) Electrode implantation in the human body. Bull Prosthet Res Spring: 51-150.
[5] Heller A, Hutchens JO, Kirby ML, Karapas F, Fernandez C (1979) Stereotaxic electrode placement in the neonatal rat. J Neurosci Meth 1(1): 41-76.
[6] Cox DD, Papanastassiou AM, Oreper D, Andken BB, Dicarlo JJ (2008) High-resolution three-dimensional microelectrode brain mapping using stereo microfocal X-ray imaging. J Neurophysiol 100(5): 2966-2976.
[7] Johnson DA, Poplawsky A, Lancaster J, Jackson R (1974) Techniques and problems in the stereotaxic placement of subcortical lesions in infant rats. Physiol Behav 13(3): 465-470.
[8] Polikov VS, Tresco PA, Reichert WM (2005) Response of brain tissue to chronically implanted neural electrodes. J Neurosci Meth 148(1):1-18.
[9] Spence AJ, Neeves KB, Murphy D, Sponberg S, Land BR et al. (2007) Flexible multielectrodes can resolve multiple muscles in an insect appendage. J Neurosci Meth 159(1): 116-124.
[10] Rodger DC, Fong AJ, Li W, Ameri H, Ahuja A, et al. (2008) Flexible parylene-based multielectrode array technology for high-density neural stimulation and recording. Sens Actuators B: Chem 132(2): 449–460.
[11] Metallo C, White RD, Trimmer BA (2011) Flexible parylene-based microelectrode arrays for high resolution EMG recordings in freely moving small animals. J Neurosci Meth 195(2): 176-184.
[12] Wu E, Im M, Yoon E (2011) A flexible fish-bone-shaped neural probe strengthened by biodegradable silk coating for enhanced biocompatibility. 16th International Solid-State Sensors, Actuators and Microsystems Conference (TRANSDUCERS), 966-969, 5-9.
[13] Lee K-K, He J, Singh A, Massia S, Ehteshami G, et al. (2004) Polyimide-based intracortical neural implant with improved structural stiffness. J Micromech Microeng 14 32.
[14] O'Brien DP, Nichols TR, Allen MG (2001) Flexible microelectrode arrays with integrated insertion devices. The 14th IEEE International Conference on Micro Electro Mechanical Systems (MEMS) 216-219, 25-25.
[15] Felix S, Shah K, George D, Tolosa V, Tooker A, et al. (2012) Removable silicon insertion stiffeners for neural probes using polyethylene glycol as a biodissolvable adhesive. Conf Proc IEEE Eng Med Biol Soc 2012: 871-874.
[16] Tien LW, Wu F, Tang-Schomer MD, Yoon E, Omenetto FG, et al. (2013) Silk as a Multifunctional Biomaterial Substrate for Reduced Glial Scarring around Brain-Penetrating Electrodes. Adv Funct Mater 23: 3185–3193.
[17] Crampon MA, Brailovski V, Sawan M, Trochu F (2002) Nerve cuff electrode with shape memory alloy armature: design and fabrication. Biomed Mater Eng 12(4): 397-410.
[18] Freed LE, Guilak F, Guo XE, Gray ML, Tranquillo R, et al. (2006) Advanced tools for
tissue engineering: scaffolds, bioreactors, and signaling. Tissue Eng 12(12):3285-3305.
[19] Tang-Schomer MD, Hu X, Tupaj M, Tien LW, Whalen M, et al. (2014) Film-based Implants for Supporting Neuron-Electrode Integrated Interfaces for The Brain. Adv Funct Mater 24(13):1938-1948.
[20] Tao H, Hwang SW, Marelli B, An B, Moreau JE, et al. (2014) Silk-based resorbable electronic devices for remotely controlled therapy and in vivo infection abatement. Proc Natl Acad Sci 111(49):17385-17389
[21] Altman GH, Diaz F, Jakuba C, Calabro T, Horan RL, et al. (2003) Silk-based biomaterials. Biomaterials 24(3): 401-416.
[22] Meinel L, Hofmann S, Karageorgiou V, Kirker-Head C, McCool J, et al. (2005) The inflammatory responses to silk films in vitro and in vivo. Biomaterials 26(2): 147-155.
[23] Omenetto FG, Kaplan DL (2010) New Opportunities for an Ancient Material. Science 329(5991): 528-531.
[24] Horan RL, Antle K, Collette AL, Wang Y, Huang J, et al. (2005) In vitro degradation of silk fibroin. Biomaterials 26(17): 3385-3393.
[25] Cao Y, Wang B (2009) Biodegradation of silk biomaterials. Int J Mol Sci 10(4): 1514-24.
[26] Belanger JH, Trimmer BA (2000) Combined kinematic and electromyographic analyses of proleg function during crawling by the caterpillar Manduca sexta. J Comp Physiol A 186(11): 1031-1039.
[27] Bell RA, Joachim FA (1978) Techniques for rearing laboratory colonies of tobacco hornworms and pink bollworms. Ann Entomol Soc Am 69: 365-373.
[28] Rockwood DN, Preda RC, Yucel T, Wang X, Lovett ML, et al. (2011) Materials fabrication from Bombyx mori silk fibroin. Nat Protoc 6(10): 1612-31.
[29] Jin HJ, Park J, Karageorgiou V, Kim UJ, Valluzzi R, et al. (2005) Water-stable silk films with reduced β-sheet content. Advanced Functional Materials 15(8): 1241-1247.
[30] Hu X, Shmelev K, Sun L, Gil ES, Park SH, et al. (2011) Regulation of silk material structure by temperature-controlled water vapor annealing. Biomacromolecules 12: 1686-1696.
[31] Trimmer BA, Weeks JC (1989) Effects of nicotinic and muscarinic agents on an identified motoneurone and its direct afferent inputs in larval Manduca sexta. J Exp Biol 144: 303-337.
[32] Wenk E, Merkle HP, Meinel L (2011) Silk fibroin as a vehicle for drug delivery applications. J Control Release 150(2):128-41.
[33] Lu S, Wang X, Qiang L, Xiaohui Z, Kluge J, et al. (2010) Insoluble and Flexible Silk Films Containing Glycerol. Biomacromolecules 11(1): 143–150.