Extraordinary variance in meta-analysis of venom toxicity of 160 most lethal ophidians and guidelines for estimating human lethal dose range
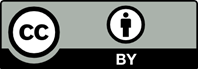
Background: This is the first meta-analysis to characterize intra-ophidian-species variation in whole venom. Being the largest possible meta-analysis at this time, it encompasses all known records of animal lethality studies over the past 100 years. These results were not artifacts of resistant test-animal species and showed orders of magnitude beyond the 1.6 logs (40-fold change) range of lethal dose documented in the literature between amphibians, lizards, and mice. Methods: A total of 1003 lethal dose study results for 160 of the most lethal venomous ophidian species in the world were analyzed. Results: LDLo was not different from LD50 across studies, indicating the true range of toxicity is probably larger. The belief that, for the route of inoculation, IC < IV < IP < IM < SC was well supported (R2 = 0.90). However, 5% of ICs were the highest dose, and 7% of SC inoculations were the lowest dose. Within the mouse test species, for one route of inoculation, the widest LD range was 2.96 logs (917-fold change, N = 20). Within mouse species, for multiple routes of inoculation, the widest LD range was 3.6 logs (4,150-fold change, N = 20). The strongest correlation for the range of lethal dose results was the number of studies (R2 = 0.56), followed by the number of test-animal species (R2 = 0.55) and then the number of routes of inoculation (R2 = 0.43). Conclusion: Scientists working with humans should use combined LDLo and LD50 meta-datasets for all data and calculate mean, median, minimum, range, and standard deviation as shown in the supplement spreadsheet, and the equations we provide. Standard deviation multiples may provide the desired safety for experimenters. For estimating the LD50 range and minimum lethal dose for species with little data, we recommend curating a meta-dataset of related snakes, and computational research to strengthen this estimation.
- Munshi H, Gajbhiye RK. Strengthening global snakebite data for WHO’s goal for 2030. Lancet. 2024;403:907-908. doi: 10.1016/S0140-6736(23)01698-7
- Hanley BP, Bains W, Church G. Review of scientific self-experimentation: Ethics history, regulation, scenarios, and views among ethics committees and prominent scientists. Rejuvenation Res. 2019;22:31-42. doi: 10.1089/rej.2018.2059
- Fry BG. Most Venomous. Wayback Machine; 2005. Available from: https://web.archive.org/ web/20140419012422/www.venomdoc.com/forums/ viewtopic.php?t=1212&postdays=0&postorder=asc& highlight=inland+taipan&start=0
- Chippaux JP, Williams V, White J. Snake venom variability: Methods of study, results and interpretation. Toxicon. 1991;29:1279-1303. doi: 10.1016/0041-0101(91)90116-9
- Calvete JJ, Sanz L, Angulo Y, Lomonte B, Gutierrez JM. Venoms, venomics, antivenomics. FEBS Lett. 2009;583:1736-1743. doi: 10.1016/j.febslet.2009.03.029
- Fernandez J, Vargas-Vargas N, Pla D, et al. Snake venomics of Micrurus alleni and Micrurus mosquitensis from the Caribbean region of Costa Rica reveals two divergent compositional patterns in New World elapids. Toxicon. 2015;107:217-233. doi: 10.1016/j.toxicon.2015.08.016
- Lomonte B, Fernandez J, Sanz L, et al. Venomous snakes of Costa Rica: Biological and medical implications of their venom proteomic profiles analyzed through the strategy of snake venomics. J Proteomics. 2014;105:323-339. doi: 10.1016/j.jprot.2014.02.020
- Sunagar K, Undheim EAB, Scheib H, et al. Intraspecific venom variation in the medically significant Southern Pacific Rattlesnake (Crotalus oreganus helleri): Biodiscovery, clinical and evolutionary implications. J Proteomics. 2014;99:68-83. doi: 10.1016/j.jprot.2014.01.013
- Creer S, Malhotra A, Thorpe RS, Stocklin RS, Favreau PS, Hao Chou WS. Genetic and ecological correlates of intraspecific variation in pitviper venom composition detected using matrix-assisted laser desorption time-of-flight mass spectrometry (MALDI-TOF-MS) and isoelectric focusing. J Mol Evol. 2003;56:317-329. doi: 10.1007/s00239-002-2403-4
- Glenn JL, Straight RC, Wolfe MC, Hardy DL. Geographical variation in Crotalus scutulatus scutulatus (Mojave rattlesnake) venom properties. Toxicon. 1983;21:119-130. doi: 10.1016/0041-0101(83)90055-7
- Poran NS, Coss RG, Benjamini E. Resistance of California ground squirrels (Spermophilus beecheyi) to the venom of the northern Pacific rattlesnake (Crotalus viridis oreganus): A study of adaptive variation. Toxicon. 1987;25:767-777. doi: 10.1016/0041-0101(87)90127-9
- Borja M, Neri-Castro E, Castañeda-Gaytán G, et al. Biological and proteolytic variation in the Venom of Crotalus scutulatus scutulatus from Mexico. Toxins (Basel). 2018;10:35. doi: 10.3390/toxins10010035
- Glenn JL, Straight RC, Wolt TB. Regional variation in the presence of canebrake toxin in Crotalus horridus venom. Comp Biochem Physiol Pharmacol Toxicol Endocrinol. 1994;107:337-346. doi: 10.1016/1367-8280(94)90059-0
- Williams V, White J, Schwaner TD, Sparrow A. Variation in venom proteins from isolated populations of tiger snakes1988;26:1067-1075. doi: 10.1016/0041-0101(88)90205-X
- Yang CC, Chang LS, Wu FS. Venom constituents of Notechis scutatus scutatus (Australian tiger snake) from differing geographic regions. Toxicon. 1991;29:1337-1344. doi: 10.1016/0041-0101(91)90120-G
- Daltry JC, Wüster W, Thorpe RS. Diet and snake venom evolution. Nature. 1996;379:537-540. doi: 10.1038/379537a0
- Alape-Giron A, Sanz L, Escolano J, et al. Snake venomics of the lancehead pitviper Bothrops asper: Geographic, individual, and ontogenetic variations. J Proteome Res. 2008;7:3556-3571. doi: 10.1021/pr800332p
- Daltry JC, Ponnudurai G, Shin CK, Tan NH, Thorpe RS, Wolfgang W. Electrophoretic profiles and biological activities: Intraspecific variation in the venom of the Malayan pit viper (Calloselasma rhodostoma). Toxicon. 1996;34:67-79. doi: 10.1016/0041-0101(95)00122-0
- Gibbs HL, Sanz L, Chiucchi JE, Farrell TM, Calvete JJ. Proteomic analysis of ontogenetic and diet-related changes in venom composition of juvenile and adult Dusky Pigmy rattlesnakes (Sistrurus miliarius barbouri). J Proteomics. 2011;74:2169-2179. doi: 10.1016/j.jprot.2011.06.013
- Durban J, Pérez A, Sanz L, et al. Integrated “omics” profiling indicates that miRNAs are modulators of the ontogenetic venom composition shift in the Central American rattlesnake, Crotalus simus simus. BMC Genomics. 2013;14:234. doi: 10.1186/1471-2164-14-234
- Margres MJ, McGivern JJ, Seavy M, Wray KP, Facente J, Rokyta DR. Contrasting modes and tempos of venom expression evolution in two snake species. Genetics. 2015;199:165-176. doi: 10.1534/genetics.114.172437
- Margres MJ, Wray KP, Seavy M, McGivern JJ, Sanader D, Rokyta DR. Phenotypic integration in the feeding system of the eastern diamondback rattlesnake (Crotalus adamanteus). Mol Ecol. 2015;24:3405-3420. doi: 10.1111/mec.13240
- Biardi JE, Chien DC, Coss RG. California ground squirrel (Spermophilus beecheyi) defenses against rattlesnake venom digestive and hemostatic toxins. J Chem Ecol. 2006;32:137-154.doi: 10.1007/s10886-006-9357-8
- Voss RS, Jansa SA. Snake-venom resistance as a mammalian trophic adaptation: Lessons from didelphid marsupials. Biol Rev Camb Philos Soc. 2012;87:822-837. doi: 10.1111/j.1469-185X.2012.00222.x
- Drabeck DH, Dean AM, Jansa SA. Why the honey badger don’t care: Convergent evolution of venom-targeted nicotinic acetylcholine receptors in mammals that survive venomous snake bites. Toxicon. 2015;99:68-72. doi: 10.1016/j.toxicon.2015.03.007
- Hayes W, Herbert S, Curtis Rehling GF, Gennaro J. Factors that influence venom expenditure in viperids and other snake species during predatory and defensive contexts. In: Schuett GW, Hoggren M, Douglas ME, Greene HW, editors. Biology of the Vipers. Eagle Mountain, Utah: Eagle Mountain Publishing; 2002. p. 207-233. Available from: http:// eaglemountainpublishing.s3.amazonaws.com/PDF/Biology of the Vipers/CH 13_hayes_.pdf
- Gibbs HL, Mackessy SP. Functional basis of a molecular adaptation: Prey-specific toxic effects of venom from Sistrurus rattlesnakes. Toxicon. 2009;53:672-679. doi: 10.1016/j.toxicon.2009.01.034
- Steinhoff S. LD50 of Venomous Snakes. 2017. Available from: https://snakedb.org/. [Last accessed on 2024 Oct 16].
- Staff. Drug Future Chemical Toxicity Database. 2017. Available from: https://www.drugfuture.com/toxic
- Linares MÁC, María R. An empirical examination of the relationship between wages and education. In: Cerrillo JCP, editor. Grau en Economia; Castelló de la Plana. Spain: Universitat Jaume; 2015. p. 22.
- Begley CG, Ellis LM. Raise standards for preclinical cancer research. Nature. 2012;483:531-533. doi: 10.1038/483531a
- Roberts TE, Bridge TC, Caley MJ, Baird AH. The point count transect method for estimates of biodiversity on coral reefs: Improving the sampling of rare species. PLoS One. 2016;11:e0152335. doi: 10.1371/journal.pone.0152335
- Chao A, Chiu CH, Hsieh TC, Davis T, Nipperess DA, Faith DP. Rarefaction and extrapolation of phylogenetic diversity. Methods Ecol Evol. 2015;6:380-388. doi: 10.1111/2041-210X.12247
- NIST/SEMATECH. F-Distribution. In: e-Handbook of Statistical Methods. Gaithersburg, MD: National Institute of Standards and Technology; 2012. Available from: https://www. itl.nist.gov/div898/handbook/eda/section3/eda3665.htm [Last accessed on 2024 Oct 17].
- Katshu MZ, Dubey I, Khess CRJ, Sarkhel S. Snake bite as a novel form of substance abuse: Personality profiles and cultural perspectives. Subst Abuse. 2011;32:43-46. doi: 10.1080/08897077.2011.540482
- Senthilkumaran S, Shah S, Balamurugan N, Menezes RG, Thirumalaikolundusubramanian P. Repeated snake bite for recreation: Mechanisms and implications. Int J Crit Illn Inj Sci. 2013;3:214-216. doi: 10.4103/2229-5151.119202